CellR4 2014; 2 (4): e1109
Impact of Immunosuppressive Drugs on Rat Beta Cell Proliferation
Topic: Cell Differentiation
Category: Original Articles
Abstract
INTRODUCTION: Despite improvement of the functional results of islet transplantation, some attrition of graft function is still observed over the years. The reason is undefined to date but is likely to be multifactorial, one factor being the adverse effects of immunosuppressive agents. In this study, we examined the effects of various immunosuppressive drugs used in clinical islet transplantation on beta cell replication in vitro.
MATERIALS AND METHODS: Islets isolated from rat pancreas were dispersed into single cells and plated on extracellular matrix. Cells were incubated in the presence of 10 microM Ethinyl deoxyUridine (EdU), with or without cyclosporine A (CsA), tacrolimus and mycophenolic acid (MPA). Dose-response and time-dependence curves were determined for each drug. Cells were labelled bydouble immunofluorescence for PDX1 and EdU, and the rate of replication expressed as the percentage of EdU positive beta cells.
RESULTS: MPA, tacrolimus, and CsA reduced glucose-stimulated insulin secretion. After 2 days of treatment, MPA inhibited cell proliferation in a dose-dependent manner with a significant effect already at 1 microg/ml. Treatment with CsA (200 ng/ml) and tacrolimus (10 ng/ml) during 5 days significantly reduced islet cell proliferation by 78% as compared to control. None of the immunosuppressive drugs induced a significant increase of apoptosis. Importantly, the effect of immunosuppressive drugs on cell proliferation was reversible.
CONCLUSIONS: Our results indicate that immunosuppressive drugs, at plasma-drug concentrations usually used to prevent islet graft rejection, inhibit beta cell proliferation. These data suggest that islet graft attrition may result in part from an impairment of beta cell regeneration induced by immunosuppressive drugs.
INTRODUCTION
Islet transplantation has become a well-accepted therapy for patients with type 1 diabetes 1 , 2. Key elements for this success include sufficient transplanted islet cell mass and adapted immunosuppressive protocols. The Edmonton protocol and its more recent variants require lifelong immunosuppression to prevent islet graft rejection 3 , 4. These protocols use a glucocorticoid-free combination of immunosuppressive drugs typically including T-cell targeting induction, and a combination of calcineurin inhibitors, mTOR inhibitors or anti-metabolites. With the original Edmonton immunosuppressive regimen, associating daclizumab, sirolimus and tacrolimus, only 20% of patients have remained insulin-independent three years after islet transplantation 5. More efficient immunosuppressive protocols have allowed a marked improvement of clinical outcomes 6 , 7, indicating that immune mechanisms were partly responsible for progressive graft dysfunction. However, functional attrition of islet grafts is still observed and additional mechanisms may be, at least in part, the result of adverse effects of immunosuppressive agents.
Tacrolimus (FK506) is a hydrophobic macrocyclic lactone, and, as cyclosporine A (CsA) inhibits calcineurin (phosphoprotein phosphatase-2B) activity and suppresses interleukin (IL)-2 transcription and T-cell proliferation 8. Combination of these immunosuppressive agents provides great immunologic protection without severe diabetogenic side effects in the short term 9. Other immunosuppressive drugs, such as mycophenolate mofetil (MMF) are increasingly utilized in islet transplantation. In a report, MMF was designed as the ideal immunosuppressive agent from a pancreatici beta cell point of view 10. Mycophenolate mofetil is a prodrug of mycophenolic acid (MPA) that was developed to improve the bioavailability of MPA. After oral administration, MMF is completely metabolized to MPA. MPA is a reversible inhibitor of inosine monophosphate dehydrogenase (IMPDH), which blocks lymphocyte proliferation 11.
Several studies have investigated the effects of these immunosuppressive agents on beta cell function and viability in cell lines, rodent and human islets 12 , 13 , 14 , 15. High doses of tacrolimus caused an acute insulin release 16 and inhibition of insulin secretion upon stimulation by glucose 17. MPA reduces insulin secretion by inhibiting voltage-dependent calcium channels 18.
Evidence indicates that beta cells in adult mammals are normally derived and replenished through self-replication 19 , 20. To achieve homeostasis in conditions of changing insulin demand, the ability of beta cells to up-or down-regulate their mass using mechanisms of beta-cell replication, neogenesis, apoptotic cell death, and changes in beta cellsize has also been demonstrated 21 , 22. Therefore beta cell replication is thought to play a quantitatively significant role in maintaining pancreatic beta cell mass and function, and the endocrine pancreas should be considered as a slowly regenerating tissue 23.
Abundant, and sometimes contradictory, literature is available on the effects of sirolimus on beta-cell function, viability and proliferation 24. We have already published an in-depth analysis on the dose- and time-dependence of sirolimus on beta cell proliferation 25. Despite the considerable interest for the effect of these drugs on islet function and viability, little is known about the effect of tacrolimus, CsA and MPA on beta cell replication. In this study, we have investigated in vitro the impact of immunosuppressive drugs on rat beta-cell proliferation.
MATERIALS AND METHODS
Immunosuppressive Agents and Antibodies
CsA, tacrolimus, and MPA were purchased from Sigma-Aldrich (Buchs, Switzerland). For immunofluorescence, primary antibody was rabbit anti-PDX1 (A kind gift from C. Wright, Nashville, TN) and secondary antibody was Alexa fluor 555-conjugated anti-rabbit (Molecular Probes, Eugene, OR). Hoechst 33342 was purchased from Sigma-Aldrich (Buchs, Switzerland).
Animals
Male Sprague-Dawley rats (200-225 g) were purchased from Janvier (Le Genest-St-Isle, France). Animals were kept in our animal facility with unrestricted access to food and water. All experiments were conducted under protocols reviewed and approved by our institutional animal care and use committee.
Rat Islet Isolation
Islets of Langerhans were isolated by collagenase digestion of rat pancreases, followed by Ficoll purification using a method routinely used in our laboratory 26 Islets were trypsinized and cells were washed twice in 10-15 ml of sterile DMEM (Gibco, Invitrogen, Basel, Switzerland) containing 10% fetal calf serum, 11.2 mM glucose, 110 µg/mlsodium pyruvate and supplemented with 110 units/ml penicillin,110 µg/ml streptomycin, and 50 µg/ml gentamycin, followed by centrifugation for 10 min at 130 g. Aliquots of 3 x 105 cells were seeded in non-adherent 100-mm diameter Petri dishes containing 9 ml of medium and incubated for 20 h at 37°C to allow cell recovery.
Cell Culture
After cell recovery, islet cells were re-suspended at a density of 4 x 105 cells/ml in control DMEM. Aliquots (50 µl) of this suspension were plated as droplets at the center of Petri dishes coatedwith 804G-ECM as previously described 27 and were incubated at 37°C. One day later, cells were incubated with 10 mM 5-ethynyl-2’-deoxyuridine (EdU) under control conditions or in continuous presence of the immunosuppressive drugs at the indicated concentrations and times. The inhibitors were dissolved in DMSO, which was added at the same concentration to control cultured cells.
Static Insulin Secretion
Islet cells were washed 3x with Krebs-Ringer bicarbonate HEPES buffer (KRBH: 125 mM NaCl, 4.74 mM KCl, 1 mM CaCl2, 1.2 mM KH2PO4, 1.2 mM MgSO4, 5 mM NaHCO3, 25 mM HEPES, pH 7.4, 0.1% BSA) containing 2.8 mM glucose and preincubated 1 h at 37°C with KRBH containing 2.8 mM glucose, followed by successive 1h incubations at 37°C in KRBH with 2.8 and 16.7 mM glucose. Insulin was extracted from cells with acid-ethanol, and insulin in buffers and extracts measured by ELISA (Mercodia, Uppsala, Sweden) with rat or insulin as the standard. Total insulin is the sum of cellular insulin and insulin secreted during the first and second incubation periods.
Detection of Proliferation
Cell replication in vitro was determined by EdU incorporation and beta-cells identified by PDX1 immunofluorescence. Islet cells incubated with EdU were fixed (10% neutral buffered methanol-free formalin, 30 min room temperature), permeabilized (0.1% Triton X-100, 15 min), and incorporated EdU estimated using a histochemical assay kit as describedby the manufacturer (Click-iT® EdU Cell Proliferation Assays, Invitrogen, Basel, Switzerland). To label PDX1, cells were exposed 2h with an anti-PDX1 antibody (1/1000). Following extensive washing, cells were incubated with Alexa fluor 555-conjugated anti-rabbit Ig. Nuclei were stained with 10 µg/mlHoechst 33342. The percentage of EdU-positive cells among PDX1 positive cells was calculated.
Apoptosis Assessment
Terminal deoxynucleotide transferase mediated dUTP nick-end labeling (TUNEL) staining on fixed cells or on tissue sections were performed with the In Situ Cell Death Detection Kit (Roche, Rotkeuz, Switzerland) following manufacturer’s instructions. Cells were stained for 20 min at 37°C with 1 μg/ml Hoechst 33342 and the number of TUNEL positive cells was expressed relative to the number of Hoechst positive cells.
The quantification of mono- and oligonucleosomes present in the cytoplasm of apoptotic cells was performed by enzyme-linked immunosorbent assay (ELISA) using the cell death detection ELISAPLUS kit according to the manufacturer’s instructions (Roche, Rotkeuz, Switzerland).
Presentation of Data and Statistical Analysis
Unless statedotherwise, data are presented as mean ± SE for “n” independent experiments, and levels of significance for differences between means were assessed either by Student’s t test or ANOVA with Dunnett post hoc analysis wherever applicable. Differences between groups were considered statistically significant at p < 0.05.
RESULTS
Immunosuppressive Drugs Decrease Glucose-Induced Iinsulin Secretion
Rat islet cells cultured with one of the different immunosuppressive drugs for 2 days were submitted to a static insulin secretion test. Glucose-induced insulin secretion was significantly reduced by tacrolimus (10 ng/ml) and MPA (5 mg/ml) (Figure 1 A). After 5 days of culture, CsA (200 ng/ml) also decreased glucose stimulated insulin secretion (Figure 1 B).
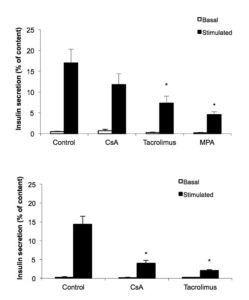
Figure 1. Immunosuppressive drugs decrease glucose-stimulated insulin secretion. Rat islet cells were attached on culture dishes during 24h. Then, cells were exposed or not to the indicated immunosuppressive drugs (200 ng/ml CsA; 10 ng/ml tacrolimus and 5 μg/ml MPA). After 2 days (A) or 5 days (B), insulin secretion was assessed in response to 2.8 mM glucose (open bars) and 16.7 mM glucose (filled bars). Mean ± SEM, n=3-5; *p < 0.05 vs. control.
Immunosuppressive Drugs Did Not Induced Apoptosis
Islet cells were exposed or not to immunosuppressive drugs for 2 or 7 days and apoptosis analysed first by TUNEL (Figure 2 A). Under control conditions, 0.2% of beta cells were TUNEL positive. Exposition to the immunosuppressive drugs did not significantly affect this low rate of apoptosis. Apoptosis was also measured by ELISA (Figure 2B). The apoptotic index was similar between control and immunosuppressive drug-treated cells, indicating that each of the tested immunosuppressive drugs was without effect on apoptosis at the concentrations used.
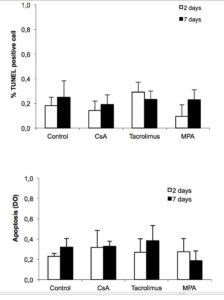
Figure 2. Immunosuppressive drugs do not affect beta cell survival. Rat islet cells were attached on culture dishes during 24h. Then, cells were exposed or not to the indicated immunosuppressive drugs (200 ng/ml CsA; 10 ng/ml tacrolimus and 5 μg/ml MPA). After 2 or 7 days, apoptosis was determined by TUNEL immunofluorescence (A) or in total islet cells by Cell Death detection ELISA (B). Mean ± SEM, n=3-5; *p < 0.05 vs. control.
Immunosuppressive Drugs Decrease Beta Cell Replication
To study the impact of immunosuppressive drugs on beta cell replication, rat islet cells were incubated with EdU in the presence or absence of CsA, tacrolimus or MPA. Cell replication was determined by EdU incorporation and beta-cells identified by PDX1 immunofluorescence. After 1, 2 and 5 days of culture in the presence of EdU and absence of immunosuppressive drugs, 5, 13 and 28% of beta-cells were EdU positive, respectively (Figure 3 Aa and B). When cells were incubated with MPA (5 mg/ml), CsA (200 ng/ml) or tacrolimus (10 ng/ml), fewer EdU positive cells were observed compared to control conditions (Figure 3 Ad, b, c and a respectively). Tacrolimus and CsA significantly reduced beta cell replication compared to control after 5 days and not after 1 and 2 days expositions (Figure 3 B). By contrast, MPA (5 mg/ml) significantly reduced beta cell replication compared to control already after 1 day and after 2 and 5 days expositions (Figure 3 B). The effect of MPA was dose-dependent and already significant at 1 mg/ml (Figure 3 C).
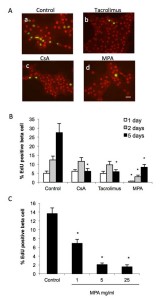
Figure 3. Immunosuppressive drugs inhibit beta cell proliferation. Islet cells were attached on culture dishes during 24h. Then cells were cultured for additional 1-5 days with EdU in presence or not of CsA, tacrolimus or MPA, at the concentration indicated. Cells were fixed and replication in beta cells was determined by EdU incorporation and PDX1 immnunofluorescence. A, fluorescence microscopic views of cells labelled for EdU (green) and PDX1 (red), bar = 20 μm. B-D, bar graphs showing the quantifications of EdU positive beta cells, mean ± SEM, n=3-5; *p < 0.05 vs. control.
Effect of Immunosuppressive Drugs on Beta-Cell Proliferation is Reversible
To test whether the inhibitory effects of immunosuppressive drugs on beta cell replication were reversible, islet cells exposed to the various immunosuppressive drugs for 2 or 5 days were cultured for another 3- or 6-day period under control conditions in presence of EdU. Removal of MPA from the culture for 3 days restored beta-cell proliferation to levels similar to control (Figure 4 A). For CsA and tacrolimus, beta-cell proliferation activity recovered within 6 days after drug removal (Figure 4 B).
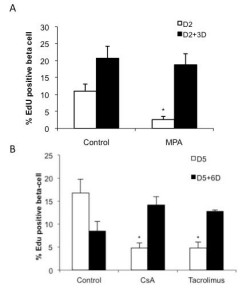
Figure 4. Effect of immunosuppressive drugs on beta cell proliferation is reversible. After attachment on culture dishes for 24h, islet cells were cultured in presence or absence of indicated immunosuppressive drugs (5 μg/ml MPA, 200 ng/ml CsA or 10 ng/ml tacrolimus) for 2 or 5 days. Then, cells were incubated in control medium with EdU for additional 3 or 6 days. Cells were fixed and replication in beta cells was determined by EdU incorporation and PDX1 immunofluorescence, mean ± SEM, n=3-5; *p < 0.05 vs. control.
DISCUSSION
In the present study, we show that the most commonly immunosuppressive drugs used in clinical islet transplantation inhibit pancreatic beta cell proliferation in vitro.
Some effects of immunosuppressive agents on rodent beta cell function and survival have been already documented 28 , 29 , 30. In this study, we confirm the deleterious effect of immunosuppressive agents on insulin secretion but not on beta cell apoptosis. This discrepancy could be due to the fact that we attached beta cells on an extracellular matrix. We have previously shown that this ECM protects beta cell from apoptosis induced by cytokines or serum deprivation 31. Whether ECM also protects beta cell from apoptosis induced by direct immunosuppressive drug toxicity remains to be investigated.
Although their chemical structure is different, CsA and tacrolimus both induce inhibition of the phosphatase activity of calcineurin 32. These data suggest that calcineurin plays an important role in beta cell proliferation and are consistent with previous studies demonstrating that calcineurin/NFAT signaling regulate pancreatic beta cell growth and function 33 , 34.
An antiproliferative effect of MMF was reported in human mesenchymal stem cells 35 and in cultured human fibroblasts 36. Most relevant to the current study, MMF has been shown to inhibit human pancreatic duct-cell proliferation 37 and DNA synthesis in pancreatic beta cell lines 38. These results also suggest that IMPDH, a rate-limiting enzyme in de novo synthesis of the purine guanosine triphosphate (GTP), and of which the two isoforms are expressed in islets 39, plays a central role in beta cell replication and maintenance of adequate islet mass.
Of importance, we show that the antiproliferative effect of immunosuppressive drugs is reversible. This indicates that immunosuppressive drugs do not cause massive beta cell death or irreversible nonspecific toxic effects. These results are in agreement with those reporting the effect of immunosuppressants on insulin secretion. Tacrolimus-induced impaired insulinsecretion was reversed 3 days after the removal of the drug 40 and the acute effects of CsA in pancreatic beta cells have been shown to be reversible 41. From a clinical perspective this observation could be relevant, since dosage and type of immunosuppressive drugs could be tailored during follow-up, depending on fluctuations of graft function.
Immunosuppressive drugs affect both insulin secretion and beta cell proliferation. A recent study has demonstrated that insulin secreted by beta cells in response to elevated glucose exerts autocrine effects on beta cell proliferation 42, suggesting that the effect of immnunosuppressive drugs on beta cell proliferation could be mediated by a decrease in insulin secretion. However, we have previously shown that immunosuppressive drugs were still able to affect beta cell proliferation when islet cells were incubated in conditions of high insulin concentration 43. These results suggest that the inhibitory effect of immunosuppressive drugs on beta cell proliferation is not due to a decrease in insulin release of treated cells.
Immunosuppressive drugs inhibit lymphocyte proliferation by targeting a number of intracellular signaling cascades that include calcineurin and IMPDH. These targets are not specific for lymphocytes and their presence in beta cells may explain the inhibitory effects of immunosuppressive drugs on insulin secretion and beta cell proliferation reported in this study.
These data were generated with rodent islets and it is legitimate to ask whether these observations are applicable to the human situation. Within the time frame of these experiments, we could not see evidence of endocrine cell proliferation in cultured human islets (data not shown). This has been a subject of debate, but it is admitted that human beta cell proliferation does occur at a lower turnover rate than in rodents, both in vivo and in vitro, possibly in relation to the extreme differences in lifespan 44. It has been also argued that, since this makes it unlikely to observe significant differences in proliferation studies using human islets in experiments with a reasonable time frame, studies performed with rodent islets are a valuable surrogate 45.
Taken together, our results suggest that progressive islet graft dysfunction may result in part from impairment of beta cell regeneration induced by immunosuppressive drugs in islet transplant recipients. These data also hint at the importance of developing alternative immunosuppressive regimens devoid of antiproliferative effects with the aim of preventing attrition of islet grafts.
Funding Sources: This work was supported by grants from the Swiss National Science Foundation (310030-143462 and 310030-149798 to DB and TB), and from the Insuleman Foundation (DB).
Duality of Interest: All authors declare that there is no duality of interest associated with this manuscript.
Acknowledgements
We thank Corinne Sinigaglia for her excellent technical assistance.
References
- Benhamou PY, Milliat-Guittard L, Wojtusciszyn A, Kessler L, Toso C, Baertschiger R, et al. Quality of life after islet transplantation: data from the GRAGIL 1 and 2 trials. Diabet Med 2009; 26(6): 617-621. (back)
- Ichii H, Ricordi C. Current status of islet cell transplantation. J Hepatobiliary Pancreat Surg 2009; 16(2): 101-112. (back)
- Berney T, Bucher P, Mathe Z, Andres A, Bosco D, Mage R, et al. Islet of Langerhans allogeneic transplantation at the University of Geneva in the steroid free era in islet after kidney and simultaneous islet-kidney transplantations. Transplant Proc 2004; 36(4): 1121-1122. (back)
- Shapiro AM, Ricordi C, Hering BJ, Auchincloss H, Lindblad R, Robertson RP, et al. International trial of the Edmonton protocol for islet transplantation. N Engl J Med 2006; 355(13): 1318-1330. (back)
- Alejandro R, Barton FB, Hering BJ, Wease S. 2008 Update from the Collaborative Islet Transplant Registry. Transplantation 2008; 86(12): 1783-1788. (back)
- Barton FB, Rickels MR, Alejandro R, Hering BJ, Wease S, Naziruddin B, et al. Improvement in outcomes of clinical islet transplantation: 1999-2010. Diabetes Care 2012; 35(7): 1436-1445. (back)
- Bellin MD, Barton FB, Heitman A, Harmon JV, Kandaswamy R, Balamurugan AN, et al. Potent induction immunotherapy promotes long-term insulin independence after islet transplantation in type 1 diabetes. Am J Transplant 2012; 12(6): 1576-1583. (back)
- Ho S, Clipstone N, Timmermann L, Northrop J, Graef I, Fiorentino D, et al. The mechanism of action of cyclosporin A and FK506. Clin Immunol Immunopathol 1996; 80(3 Pt 2): S40-45. (back)
- Nanji SA, Shapiro AM. Advances in pancreatic islet transplantation in humans. Diabetes Obes Metab 2006; 8(1): 15-25. (back)
- Polastri L, Galbiati F, Bertuzzi F, Fiorina P, Nano R, Gregori S, et al. Secretory defects induced by immunosuppressive agents on human pancreatic beta-cells. Acta Diabetol 2002; 39(4): 229-233. (back)
- Allison AC, Eugui EM. Mycophenolate mofetil and its mechanisms of action. Immunopharmacology 2000; 47(2-3): 85-118. (back)
- Polastri L, Galbiati F, Bertuzzi F, Fiorina P, Nano R, Gregori S, et al. Secretory defects induced by immunosuppressive agents on human pancreatic beta-cells. Acta Diabetol 2002; 39(4): 229-233. (back)
- Bell E, Cao X, Moibi JA, Greene SR, Young R, Trucco M, et al. Rapamycin has a deleterious effect on MIN-6 cells and rat and human islets. Diabetes 2003; 52(11): 2731-2739. (back)
- Fuhrer DK, Kobayashi M, Jiang H. Insulin release and suppression by tacrolimus, rapamycin and cyclosporin A are through regulation of the ATP-sensitive potassium channel. Diabetes Obes Metab 2001; 3(6): 393-402. (back)
- Paty BW, Harmon JS, Marsh CL, Robertson RP. Inhibitory effects of immunosuppressive drugs on insulin secretion from HIT-T15 cells and Wistar rat islets. Transplantation 2002; 73(3): 353-357. (back)
- Fuhrer DK, Kobayashi M, Jiang H. Insulin release and suppression by tacrolimus, rapamycin and cyclosporin A are through regulation of the ATP-sensitive potassium channel. Diabetes Obes Metab 2001; 3(6): 393-402. (back)
- Hui H, Khoury N, Zhao X, Balkir L, D’Amico E, Bullotta A, et al. Adenovirus-mediated XIAP gene transfer reverses the negative effects of immunosuppressive drugs on insulin secretion and cell viability of isolated human islets. Diabetes 2005; 54(2): 424-433. (back)
- Li GD, Luo RH, Metz SA. Effects of inhibitors of guanine nucleotide synthesis on membrane potential and cytosolic free Ca2+ levels in insulin-secreting cells. Biochem Pharmacol 2000; 59(5): 545-556. (back)
- Butler AE, Janson J, Bonner-Weir S, Ritzel R, Rizza RA, Butler PC. Beta-cell deficit and increased beta-cell apoptosis in humans with type 2 diabetes. Diabetes 2003; 52(1): 102-110. (back)
- Dor Y, Brown J, Martinez OI, Melton DA. Adult pancreatic beta-cells are formed by self-duplication rather than stem-cell differentiation. Nature 2004; 429(6987): 41-46. (back)
- Bernard-Kargar C, Ktorza A. Endocrine pancreas plasticity under physiological and pathological conditions. Diabetes 2001; 50 Suppl 1: S30-35. (back)
- Bonner-Weir S. Life and death of the pancreatic beta cells. Trends Endocrinol Metab 2000; 11(9): 375-378. (back)
- Finegood DT, Scaglia L, Bonner-Weir S. Dynamics of beta-cell mass in the growing rat pancreas. Estimation with a simple mathematical model. Diabetes 1995; 44(3): 249-256. (back)
- Berney T, Secchi A. Rapamycin in islet transplantation: friend or foe? Transpl Int 2009; 22(2): 153-161. (back)
- Niclauss N, Bosco D, Morel P, Giovannoni L, Berney T, Parnaud G. Rapamycin impairs proliferation of transplanted islet beta cells. Transplantation 2011; 91(7): 714-722. (back)
- Finegood DT, Scaglia L, Bonner-Weir S. Dynamics of beta-cell mass in the growing rat pancreas. Estimation with a simple mathematical model. Diabetes 1995; 44(3): 249-256. (back)
- Parnaud G, Hammar E, Ribaux P, Donath MY, Berney T, Halban PA. Signaling pathways implicated in the stimulation of beta-cell proliferation by extracellular matrix. Mol Endocrinol 2009; 23(8): 1264-1271. (back)
- Bell E, Cao X, Moibi JA, Greene SR, Young R, Trucco M, et al. Rapamycin has a deleterious effect on MIN-6 cells and rat and human islets. Diabetes 2003; 52(11): 2731-2739. (back)
- Paty BW, Harmon JS, Marsh CL, Robertson RP. Inhibitory effects of immunosuppressive drugs on insulin secretion from HIT-T15 cells and Wistar rat islets. Transplantation 2002; 73(3): 353-357. (back)
- Uchizono Y, Iwase M, Nakamura U, Sasaki N, Goto D, Iida M. Tacrolimus impairment of insulin secretion in isolated rat islets occurs at multiple distal sites in stimulus-secretion coupling. Endocrinology 2004; 145(5): 2264-2272. (back)
- 26 (back)
- Ho S, Clipstone N, Timmermann L, Northrop J, Graef I, Fiorentino D, et al. The mechanism of action of cyclosporin A and FK506. Clin Immunol Immunopathol 1996; 80(3 Pt 2): S40-45. (back)
- Parnaud G, Hammar E, Ribaux P, Donath MY, Berney T, Halban PA. Signaling pathways implicated in the stimulation of beta-cell proliferation by extracellular matrix. Mol Endocrinol 2009; 23(8): 1264-1271. (back)
- Heit JJ. Calcineurin/NFAT signaling in the beta-cell: From diabetes to new therapeutics. Bioessays 2007; 29(10): 1011-1021. (back)
- Hoogduijn MJ, Crop MJ, Korevaar SS, Peeters AM, Eijken M, Maat LP, et al. Susceptibility of human mesenchymal stem cells to tacrolimus, mycophenolic acid, and rapamycin. Transplantation 2008; 86(9): 1283-1291. (back)
- Morath C, Reuter H, Simon V, Krautkramer E, Muranyi W, Schwenger V, et al. Effects of mycophenolic acid on human fibroblast proliferation, migration and adhesion in vitro and in vivo. Am J Transplant 2008; 8(9): 1786-1797. (back)
- Gao R, Ustinov J, Korsgren O, Otonkoski T. Effects of immunosuppressive drugs on in vitro neogenesis of human islets: mycophenolate mofetil inhibits the proliferation of ductal cells. Am J Transplant 2007; 7(4): 1021-1026.0 (back)
- Metz S, Holland S, Johnson L, Espling E, Rabaglia M, Segu V, et al. Inosine-5′-monophosphate dehydrogenase is required for mitogenic competence of transformed pancreatic beta cells. Endocrinology 2001; 142(1): 193-204. (back)
- Metz S, Holland S, Johnson L, Espling E, Rabaglia M, Segu V, et al. Inosine-5′-monophosphate dehydrogenase is required for mitogenic competence of transformed pancreatic beta cells. Endocrinology 2001; 142(1): 193-204. (back)
- Uchizono Y, Iwase M, Nakamura U, Sasaki N, Goto D, Iida M. Tacrolimus impairment of insulin secretion in isolated rat islets occurs at multiple distal sites in stimulus-secretion coupling. Endocrinology 2004; 145(5): 2264-2272. (back)
- Hahn HJ, Dunger A, Laube F, Besch W, Radloff E, Kauert C, et al. Reversibility of the acute toxic effect of cyclosporin A on pancreatic B cells of Wistar rats. Diabetologia 1986; 29(8): 489-494. (back)
- Muller D, Huang GC, Amiel S, Jones PM, Persaud SJ. Identification of insulin signaling elements in human beta-cells: autocrine regulation of insulin gene expression. Diabetes 2006; 55(10): 2835-2842. (back)
- Niclauss N, Bosco D, Morel P, Giovannoni L, Berney T, Parnaud G. Rapamycin impairs proliferation of transplanted islet beta cells. Transplantation 2011; 91(7): 714-722. (back)
- Meier JJ, Butler AE, Saisho Y, Monchamp T, Galasso R, Bhushan A, et al. Beta-cell replication is the primary mechanism subserving the postnatal expansion of beta-cell mass in humans. Diabetes 2008; 57(6): 1584-1594. (back)
- Stewart AF. Betatrophin versus bitter-trophin and the elephant in the room: time for a new normal in beta-cell regeneration research. Diabetes 2014; 63(4): 1198-1199. (back)
To cite this article
Impact of Immunosuppressive Drugs on Rat Beta Cell Proliferation
CellR4 2014; 2 (4): e1109
Publication History
Published online: 26 Jul 2014

This work is licensed under a Creative Commons Attribution-NonCommercial-ShareAlike 4.0 International License.